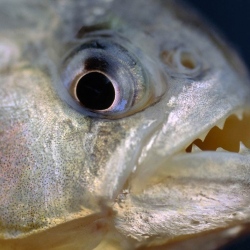
A team from Monash University’s Australian Regenerative Medicine Institute (ARMI), led by Dr Yona Goldshmit and Professor Peter Currie, discovered the role of a protein in the remarkable self-healing ability of the fish.
The findings, detailed in The Journal of Neuroscience, could eventually lead to ways to stimulate spinal cord regeneration in humans.
When the spinal cord is severed in humans and other mammals, the immune system kicks in, activating specialised cells called glia to prevent bleeding into it, Professor Currie said.
“Glia are the workmen of nervous system. The glia proliferate, forming bigger cells that span the wound site in order to prevent bleeding into it. They come in and try to sort out problems. A glial scar forms,” Professor Currie said.
However, the scar prevents axons, threadlike structures of nerve cells that carry impulses to the brain, of neighbouring nerve cells from penetrating the wound. The result is paralysis.
“The axons upstream and downstream of the lesion sites are never able to penetrate the glial scar to reform. This is a major barrier in mammalian spinal cord regeneration,” Professor Currie said.
In contrast, the zebra fish glia form a bridge that spans the injury site but allow the penetration of axons into it.
The fish can fully regenerate its spinal cord within two months of injury. “You can’t tell there’s been any wound at all,” Professor Currie said.
Scientists discovered the protein, called fibroblast growth factor (fgf), controlled the shape of the glia, and accounted for the difference in the response to spinal cord injury between humans and zebra fish.
The scientists showed the protein could be manipulated in the zebra fish to speed up tissue repair even more.
“The hope is that fgf could eventually be used to promote better results in spinal cord repair in people,” Professor Currie said.
Adult zebrafish show a remarkable capacity to regenerate their spinal column after injury, an ability that stands in stark contrast to the limited repair that occurs within the mammalian CNS post-injury.
The reasons for this interspecies difference in regenerative capacity remain unclear. Here we demonstrate a novel role for Fgf signaling during glial cell morphogenesis in promoting axonal regeneration after spinal cord injury. Zebrafish glia are induced by Fgf signaling, to form an elongated bipolar morphology that forms a bridge between the two sides of the resected spinal cord, over which regenerating axons actively migrate.
Loss of Fgf function inhibits formation of this “glial bridge” and prevents axon regeneration. Despite the poor potential for mammalian axonal regeneration, primate astrocytes activated by Fgf signaling adopt a similar morphology to that induced in zebrafish glia. This suggests that differential Fgf regulation, rather than intrinsic cell differences, underlie the distinct responses of mammalian and zebrafish glia to injury.