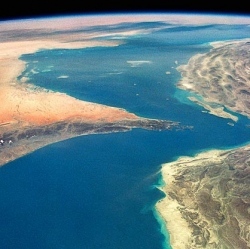
Gravity is a constant for all organisms on Earth. It acts on every aspect of our physiology, no matter what you are, you evolved in an environment where gravity roots us firmly to the ground. But what happens if you’re removed from that familiar environment and placed into a situation outside your evolutionary experience?
That’s exactly the question we ask every day of the plants we grow in our laboratory. They start out here in our earthbound lab, but they’re on their way to outer space. What could be a more novel environment for a plant than the zero-gravity conditions of spaceflight?
By studying how plants react to life in space, we can learn more about how they adapt to environmental changes. Not only are plants crucial to almost every facet of life on Earth; plants will be critical to our explorations of the universe. As we look to a future of possible space colonization, it’s vital to understand how plants will fare off planet before we rely on them within space outposts to recycle our air and water and supplement our food.
So even while we stay right here on the ground, our research plants blast off and head to the International Space Station (ISS). Already they’ve given us some surprises about growing in zero gravity—and shaken up some of our thinking about how plants grow on Earth.
Plants make especially great research subjects if you’re interested in environmental stress. Because they’re stuck in one spot, what we biologists call sessile organisms, plants must cleverly deal in place with whatever their environment throws at them. Moving to a more favorable spot isn’t an option, and they can do little to alter the environment around them.
But what they can do is alter their internal “environment”, and plants are masters of manipulating their metabolism to cope with perturbations of their surroundings. This characteristic is one of the reasons we use plants in our research; we can count on them to be sensitive reporters of environmental change, even in novel environments like spaceflight.
Folks have been curious about how plants respond to spaceflight from the very beginning of our ability to get there. We launched our first spaceflight experiment on Space Shuttle Columbia back in 1999, and the things we learned then are still fueling new hypotheses about how plants deal with the absence of gravity.
Spaceflight requires specialized growth habitats, specialized tools for observation and sample collection, and of course specialized people to take care of the experiment on orbit.
A typical experiment begins on Earth in our lab with the planting of dormant Arabidopsis seeds in Petri plates containing a nutrient gel. This gel (unlike soil) stays put in zero gravity, and provides the water and nutrients the growing plants will need. The plates are then wrapped in dark cloth, taken to Kennedy Space Center, and eventually loaded into the Dragon Capsule on top of a Falcon 9 rocket to catch a ride to the ISS.
Once docked, an astronaut inserts the plates into the plant growth hardware. The light inside stimulates the seeds to sprout, cameras record the growth of the seedlings over time, and at the end of the experiment, the astronaut harvests the 12-day-old plants and save them in tubes of preservative.
Once returned to us on Earth, we can run more tests on the preserved samples to investigate the unique metabolic processes the plants engaged while on orbit.
One of the first things we found was that certain root growth strategies that everyone had assumed need gravity actually don’t require it at all.
To seek out water and nutrients, plants need their roots to grow away from where they are planted. On Earth, gravity is the most important “cue” for the direction to grow, but plants also use touch (think of the root tip as a sensitive fingertip) to help navigate around obstacles.
Back in 1880, Charles Darwin showed that when you grow plants along a slanted surface, the roots don’t grow straight away from the seed, but rather take a jog to one side. This root growth strategy is called “skewing.” Darwin hypothesized that a combination of gravity and the root touching its way across the surface was behind it—and for 130 years, that’s what everyone else thought too.
But in 2010, we saw that the roots of the plants we grew on the ISS marched across the surface of their Petri plate in a perfect example of root skewing—no gravity required. It was quite a surprise. So what’s really behind root-skewing on orbit, since it’s obviously not gravity?
Plants on the ISS do have a potentially second source of information from which they could get a directional cue: light. We hypothesized that in the absence of gravity to point roots “away” from the direction of the leaves, light plays a bigger role in root guidance.
What we found was that yes, light is important, but not just any light will do—there has to be a gradient of light intensity for it to act as a useful guide. Think of it in terms of a good smell: you can navigate to the kitchen with your eyes closed when cookies are just coming out of the oven, but if the whole house is flooded equally with the scent of chocolate chip cookies, you couldn’t find your way.
In the absence of gravity, plants can’t use the “tools” they’re used to for navigation, so they had to craft together another solution. They can do that by regulating the way they express their genes. That way they can make more or less of specific proteins that are helpful or not in zero gravity. Various plant parts came up with their own gene regulation strategies.
We found a number of genes involved in making and remodeling cell walls are expressed differently in space-grown plants. Other genes involved with light-sensing—normally expressed in leaves on Earth—are expressed in roots on the ISS. In leaves, many genes associated with plant hormone signaling are repressed, and genes associated with insect defense are more active. These same trends are also seen in the relative abundance of proteins involved in signaling, cell wall metabolism and defense.
These patterns of genes and proteins tell a story—in microgravity, plants respond by loosening their cell walls, along with creating new ways to sense their environment.
We track these gene expression changes in real time by labeling specific proteins with a fluorescent tag. Plants engineered with glowing fluorescent proteins can then “report” how they are responding to their environment as it is happening. These engineered plants act as biological sensors—“biosensors” for short. Specialized cameras and microscopes let us follow how the plant is utilizing those fluorescent proteins.
This kind of research gives us new understanding of how plants sense and respond to external stimuli at a fundamental, molecular level. The more we can learn about how plants respond to novel and extreme environments, the more prepared we are for understanding how plants will deal with the changing environments they’re up against here on Earth.
And of course our research will inform collective efforts to take our biology off the planet. The observation that gravity isn’t as vital to plants as we once thought is welcome news for the prospect of farming on other planets with low gravity, and even on spacecraft where there is no gravity. Humans are explorers, and when we leave earth’s orbit, you can bet we’ll take plants with us!