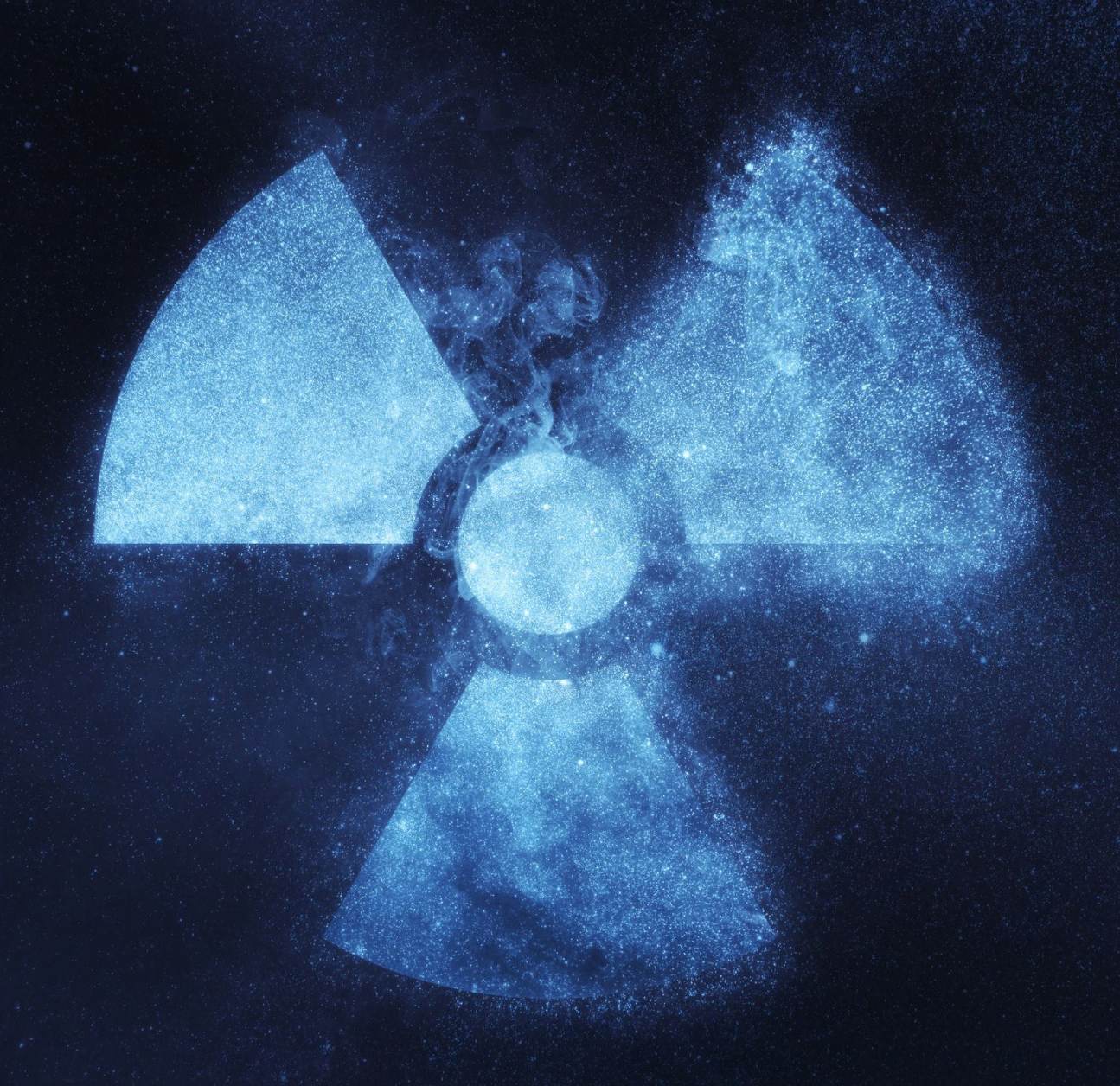
A cheap, safe, self-charging battery that delivers high power for decades without ever needing a charge? That’s a game changer. California-based company NDB is making some outrageous promises with its nano-diamond battery technology, which could completely disrupt the energy generation, distribution and provision models if deployed at scale.
Each of these batteries, which can be built to fit any existing standard or shape, uses a small amount of recycled nuclear waste, reformed into a radioactive diamond structure and coated in non-radioactive lab diamonds for safety.
Dr Nima Golsharifi: Our battery is based on the beta decay and alpha decay of radioisotopes. The technology we have encapsulates this radioisotope in a very safe manner, which allows it to be used in basically any application that current batteries are being used for.
The particular type of carbon that you’re using, where do you get that?
Nima: Basically we’re using a range of different isotopes, not just one particular one, but access to these are through different methods. We have some partners in collaboration at the moment that can provide us with them.
But they’re basically taken from nuclear waste. So we can recycle them and use the raw materials for our application. But we can also synthesize it in large scale in our facility. So both are possibilities.
What part of a nuclear reactor creates this waste? What’s it doing before it becomes waste?
Nima: Basically, some parts of the nuclear reactor, like the moderator and the refractor, are being exposed to radiation from the fuel rods. Over time they become radioactive themselves. That’s the part that they have to store as nuclear waste.
So this part could be taken away, and through some process, either gasification or some other processes we’ve designed, we can convert that into a useful raw material for our batteries.
Can you talk about how big of a waste problem that is for the nuclear industry currently?
Nima: Sure. At the moment, their expenditure is more than a hundred million dollars every year. Nuclear waste is a very large issue across the world. And beside this, there’s basically no other way to re-use it in a safe solution.
So what we’re doing covers two challenges in one. Converting nuclear waste into a battery that generates power in a very safe manner. Once this battery is used – and it can have a very long life span, it becomes a very safe byproduct that’s of no harm to the environment.
Duration depends on the type of radioisotope you’re using, and for every application the lifetime is different. But what we can say is that the battery would operate for the lifetime of the application itself, for sure. For some applications, much higher. So if you’re talking about electric vehicles, our battery could run for around 90 years without the requirement of recharging.
When it comes to something like consumer electronics, it’d be more like 9 years. In some small sensor applications, it can go for up to 28,000 years.
So what sort of quantities of this waste are there around the world? Is this super common stuff, or is it reasonably finite?
Nima: Basically we’re covering two different kinds of nuclear waste. One is intermediate, and the other is high level. So there will be a time where we have recycled the entire amount of nuclear waste, and we’ll need new solutions for the raw material. But as I mentioned, we’ll be able to produce this raw material through other methods, including transmutation.
That’s a process that’s currently being used, and not something we’ve invented ourselves. It was invented by MIT, and it involves a centrifuge to separate out the isotopes. The main ingredient is nitrogen, which is the major component of air, so it’s a very cheap solution.
How does it become safe to be used in a battery?
Nima: Basically, we can generate a high amount of cover from the radioactive substance. We’re using a combination of technologies within our structure that can make it very safe to users. Mainly it comes down to the fact that we’re using diamond structures.
Diamond itself has different interesting properties. It’s one of the best heat sinks available at the moment, for example. That on its own covers thermal safety. When it comes to mechanical safety, diamond is one of the strongest materials in the world. 11.5 times stronger than steel. So again, that itself makes the battery tamper-proof and safe.
In addition to that, we have a combination of other technologies, including the implantation of the radioisotopes within the diamond structure, which stops the spread of the radioisotopes even if the structure is broken down – which is kind of impossible without access to specific tools like lasers and others.
So in general I can say it’s a combination of technologies that we’ve either innovated or invented that create a very safe structure as a battery.
I’d like to add to that, that using radioisotopes as a source for energy is not new. We have nuclear medicine, where patients are treated with controlled equipment, which has always given effective results. Similarly, we have had nuclear-powered submarines and aircraft carriers. Of course, that’s a completely different process, but it’s been able to successfully and safely deliver power and energy without safety issues.
The choice of diamond as a material is one of the strongest natural materials, and it acts as a powerful shielding and protection mechanism.
Can you describe how the energy is extracted and harnessed?
Nima: Maybe I can give an example that could help you understand. Let’s go to solar cells, everyone’s familiar with those. These convert the energy from light radiation into electricity in photovoltaic cells.
In our case, we’re converting the radiation from alpha/beta decay – alpha and beta radiation – directly into electricity. And the mechanism we’re using is simple crystalline diamond. As I mentioned before, we have another layer, which is fully crystalline diamond, creating extra shielding and safety for this structure.
The radioactivity produced by the body is actually more than what you get from these batteries. They’re quite safe.
In terms of evaluating batteries for use in cars, eVTOLs and things like that, the main metrics seem to be energy density, power density, safety in a crash, that sort of thing.
Do you know what sort of figures you’re looking at with these batteries?
Nima: When it comes to energy density, the energy density of a basic radioisotope is far beyond anything else on the market.
When it comes to power density, the solution we have will give a higher level. But compared to the way that energy density is higher, power density is not that much higher. But it’s still significantly better than other batteries in the market.
And as far as crashes, no crash could break down our structure at all. Because you’re using the diamond, and the specific mechanisms that make it stronger. The only way to get through the structure we have is the use of specific tools and lasers, which are quite expensive.
Another way to look at this is to think of it in an iPhone. With the same size battery, it would charge your battery from zero to full, five times an hour. Imagine that. Imagine a world where you wouldn’t have to charge your battery at all for the day. Now imagine for the week, for the month… How about for decades? That’s what we’re able to do with this technology.
It would strike at the heart of the disposable model the phone companies tend to use?
You’ve hit the nail on the head there. A couple of things. One is the ability for us to power things at scale. We can start at the nanoscale and go up to power satellites, locomotives… Imagine that.
Secondly, we’re taking something that’s a big negative – radioactive waste, very dangerous – and turning it into something productive that provides electricity.
The third thing is that we wanna use this technology to get low-cost electricity to places that need it. We’ve now disrupted the whole mechanism of the creation and storage of power. There’s a lot of infrastructure needed before you can flip a light switch and a light comes on.
But with what we’ve created, you don’t need that infrastructure. You could put one of these batteries in a home, and boom, you’ve eliminated the whole infrastructure. Imagine the disruption that’s gonna cause, for good or for bad. It’ll upset a few people.
We’ve taken something that’s really harmful to the environment, a problem, and created energy. And for places that don’t have the electrical infrastructure in place, we want to provide that at a very low cost.
The nano diamond battery has the potential to totally upend the energy equation since it never needs charging and lasts many, many years.
Let’s talk about cost a little. Obviously lithium batteries cost a lot, they’re a primary component of the cost of electric vehicles. Do you guys have a sense for what these things could cost in a commercial environment?
Yes, we’ve done financial modelling around this. A lot of applications have been considered. What we can say is it’ll depend on the application, but it should be at a good competition level with current lithium-ion batteries.
In some cases, you’re a little bit higher in price for production, and in others, when it goes to scale, we’re a cheaper solution. Let me give you an example. Take the battery for a Tesla car, it costs somewhere in the region of US$9-10K. Our battery will cost something in the region of US$7-8K. But it’s different in different applications.
So, cheaper and it never needs charging, and it lasts for vastly longer than any lithium cell.
Not only is it a few thousand cheaper for the battery pack, but ours recharges itself. So on a Tesla, you need to recharge, stop, over time the battery wears itself out. Ours lasts for a long time.
We’ll probably have them available under some sort of subscription model, pay as you go, but it’ll be substantially cheaper than what the mechanism is today for a Tesla car.
Extraordinary. How far along is this technology? How far are we off mass production? Where are you at with prototyping and testing?
Nima: We’re in the prototyping stage at the moment. We’ve completed the proof of concept, and we’re about to start the commercial prototype. However, the pandemic has happened, and the lab has been shut down for some time.
But basically once the laboratories are open, we do require around 6-9 months to complete our commercial prototype, and following that to go through the regulatory process, to bring the first few applications for the battery into the market in less than two years’ time.